What profound effects does intense heat have? A deep exploration of thermal phenomena.
The concept refers to exceptionally high temperatures, extending beyond the typical range of comfortable warmth. This intense heat can manifest in various contexts, from the core of a star to the interior of a geyser. Examples encompass the extreme heat generated by volcanic activity, the scorching temperatures of a desert during a heat wave, or even the high temperatures used in industrial processes such as smelting or manufacturing. The defining characteristic is the pronounced and significant degree of heat involved.
The significance of intense heat varies across disciplines. In geological studies, understanding deep subterranean heat is crucial for comprehending plate tectonics and volcanic activity. In materials science, extreme heat facilitates the processing and shaping of metals and other substances. From a biological perspective, high temperatures can denature proteins and enzymes, potentially harming living organisms. A thorough understanding of this phenomenon is critical for safety considerations and for advanced technological applications. Historically, cultures across the globe have relied on fire and heat sources for survival and development, emphasizing its importance in human history.
Moving forward, this discussion will explore specific applications of extreme heat in different scientific and industrial contexts, examining the technologies, methods, and potential impact of working with this phenomenon.
Deep Hot
Understanding "deep hot" necessitates exploring its various facets, from extreme temperatures to their applications. Analyzing these components offers a comprehensive perspective.
- Intense heat
- Thermal properties
- Geological processes
- Industrial applications
- Material effects
- Safety protocols
- Scientific investigation
These key aspects interconnect to provide a holistic view. Intense heat, a fundamental component, dictates thermal properties and impacts geological formations. This, in turn, fuels industrial applications, requiring material science analysis and stringent safety protocols. Scientific investigation becomes crucial to understanding the processes and applications related to "deep hot," which could range from exploring the interiors of stars to manufacturing advanced materials.
1. Intense Heat
The relationship between "intense heat" and "deep hot" is fundamental. "Intense heat" represents the high degree of thermal energy, while "deep hot" signifies the concentration or manifestation of this energy in specific contexts. Intense heat is a prerequisite for deep hot; without sufficient intensity, the effect is not "deep." This connection is evident in natural phenomena like volcanic eruptions, where intense subterranean heat drives the molten rock upward. Further, the intense heat generated in a nuclear reactor core is essential for the controlled nuclear chain reaction, highlighting the profound impact of intense heat on various processes.
The importance of understanding "intense heat" within the context of "deep hot" is considerable. For instance, in metallurgy, precise control of intense heat is vital for refining and shaping metals. Similarly, in astrophysics, analyzing the intense heat within stars is crucial to understanding stellar evolution. The practical significance extends to safety protocols; the potential dangers associated with intense heat necessitate careful engineering considerations to prevent uncontrolled reactions and mitigate risks, including industrial accidents and environmental hazards. Examples range from the design of nuclear reactors to the construction of high-temperature furnaces.
In summary, "intense heat" is a critical component of "deep hot." Its intensity dictates the magnitude and manifestation of the phenomena. Comprehending this relationship is essential for a wide range of applications, from industrial processes to scientific explorations. The challenges associated with managing and controlling intense heat are substantial, requiring sophisticated technologies and safety protocols. This understanding underscores the profound influence of intense heat across various scientific and technological domains.
2. Thermal properties
Thermal properties are intrinsic characteristics of materials that govern their response to heat. A deep understanding of these properties is essential when considering "deep hot" phenomena, as they directly impact how materials behave under extreme temperatures. The ability of a substance to conduct heat, its melting point, and its thermal expansion are crucial factors in situations where intense heat is present. For example, the thermal conductivity of rock determines how efficiently heat from the Earth's interior can be transferred to the surface, influencing volcanic activity. Similarly, the high thermal conductivity of certain metals is exploited in industrial applications involving intense heat, such as forging and smelting. Changes in thermal properties under extreme heat can also lead to phase transitions, like the melting of ice or the boiling of water. Understanding these transitions is vital for predicting and managing outcomes in various applications.
The practical significance of understanding thermal properties within the context of "deep hot" is evident across numerous disciplines. In engineering, thermal properties are critical for designing materials capable of withstanding extreme heat and pressure, such as those used in engines, power generation, and aerospace applications. In geology, understanding thermal properties is fundamental for interpreting geothermal activity, assessing heat flow from the Earth's interior, and understanding the formation of mineral deposits. In materials science, the impact of intense heat on a material's thermal properties can be used to develop novel materials with specific thermal characteristics for advanced applications, including high-temperature superconductors and heat-resistant alloys. Without this understanding, the successful design and implementation of systems operating under intense heat are problematic.
In conclusion, thermal properties are indispensable components of "deep hot." Their intricate interplay with intense heat significantly influences how materials behave and respond. Understanding these properties is paramount for predicting, mitigating, or exploiting the effects of extreme heat in diverse applications, from industrial processes to geological phenomena. The challenges in measuring and analyzing thermal properties under extreme conditions drive ongoing research, pushing the boundaries of knowledge and leading to advancements in materials science and engineering.
3. Geological Processes
Geological processes are intrinsically linked to "deep hot" through the significant influence of intense subterranean heat. This heat, often originating from radioactive decay within the Earth's interior, drives various geological activities, shaping the planet's surface and influencing its dynamics. Understanding these relationships is critical for comprehending the Earth's internal workings and the consequences of these processes.
- Magmatism and Volcanism
Substantial heat within the Earth's mantle generates magma, molten rock. This molten material rises to the surface through various geological processes, often erupting as volcanoes. The intensity of the subterranean heat directly affects the volume and frequency of volcanic activity. Examples include the formation of the Hawaiian Islands, the ongoing activity of Yellowstone National Park's supervolcano, and the devastating consequences of large-scale eruptions. Understanding these deep-seated heat sources is paramount for predicting volcanic hazards and mitigating their impacts.
- Plate Tectonics
Convective currents within the Earth's mantle, driven by temperature differentials and deep heat sources, are the primary drivers of plate tectonics. The movement of these plates, caused by mantle convection, influences mountain building, earthquakes, and the formation of ocean basins. The energy released from these geological processes originates in the deep hot interior of the Earth. The ongoing interplay between tectonic plates directly relates to the deep heat within the planet. Studying these processes is essential for understanding and predicting seismic activity and its consequences.
- Metamorphism
Intense heat and pressure within the Earth's crust, often associated with tectonic plate interactions or volcanic activity, cause existing rocks to change their physical and chemical properties. This process, known as metamorphism, transforms rocks into different types, leading to new mineral formations and altering the overall geological structure. The intensity of the deep heat and associated pressure directly influences the degree of metamorphism, making it another critical link to "deep hot" phenomena. Examples include the formation of marble from limestone and the transformation of shale into slate.
- Geothermal Systems
Areas where heat from the Earth's interior is transferred to the surface provide geothermal resources. The intensity of this deep heat source dictates the availability and extent of geothermal energy, such as steam for power generation or heated water for various applications. The efficiency of these systems relies on the "deep hot" heat source sustaining the geothermal gradient. These systems illustrate the direct connection between subsurface heat and renewable energy potential.
In conclusion, the various geological processes outlined above demonstrate a strong correlation with "deep hot." The Earth's internal heat is a driving force behind volcanism, plate tectonics, metamorphism, and geothermal activity. Understanding the intricacies of these processes, coupled with knowledge of the intensity and distribution of subsurface heat, is critical for predicting natural phenomena, mitigating risks, and utilizing renewable energy sources. The consequences of neglecting this relationship can be severe, from volcanic eruptions to significant environmental changes.
4. Industrial applications
Industrial processes often necessitate the utilization of intense heat. This reliance on "deep hot" manifests in diverse applications, spanning metallurgy, manufacturing, and energy generation. The controlled application of extreme heat in these sectors is essential for achieving desired outcomes, while careful consideration of safety protocols and material properties is crucial.
- Metallurgy and Material Processing
High temperatures are fundamental in metallurgy, impacting processes like smelting, forging, and casting. Smelting, for instance, necessitates extreme heat to separate metals from ores. Forging shapes metal through intense heat, while casting utilizes precise temperature control to create complex shapes. These processes require a profound understanding of thermal properties and the behavior of materials under extreme conditions. The resulting metals exhibit enhanced strength and durability due to the transformations induced by deep heat treatment.
- Manufacturing and Chemical Synthesis
Intense heat is vital in various manufacturing processes, including the production of ceramics, glass, and advanced materials. The high temperatures employed during these processes alter the properties of raw materials, leading to desired products. Similarly, chemical synthesis frequently relies on elevated temperatures to drive reactions and achieve specific outcomes. Control over heat input is critical for optimizing reaction kinetics and minimizing undesirable byproducts. The intricate interplay of temperature, pressure, and material properties is key for success in these applications.
- Energy Production and Conversion
Industrial energy production often involves processes requiring high temperatures. Fossil fuel combustion, a common method for generating electricity, depends on the intense heat released during the combustion process. Other processes like nuclear fission and solar thermal energy also utilize immense heat for energy generation or conversion. The efficient and safe management of extreme heat is paramount in these applications to maximize energy output and minimize environmental risks.
- Heat Treatment and Annealing
Heat treatment processes, such as annealing, are crucial for modifying the microstructure and mechanical properties of metals. Annealing involves heating metals to specific temperatures to relieve internal stresses and improve ductility or hardness. The effectiveness of these procedures depends on precise temperature control and accurate timing. The desired alteration in the material's properties directly relates to the control and application of "deep hot" processes.
In conclusion, "deep hot" plays a critical role across various industrial applications. The controlled utilization of extreme heat enables the production of high-quality materials, efficient energy generation, and enhanced manufacturing processes. However, managing the potential risks associated with intense heat is vital. The intricate relationship between material science, engineering, and the effective application of heat underscores the importance of "deep hot" in the modern industrial landscape. The ongoing advancements in understanding and applying "deep hot" principles drive industrial innovations and technological advancement.
5. Material Effects
Materials subjected to "deep hot" environments experience significant alterations in their physical and chemical properties. This response, termed "material effects," is a crucial component of understanding phenomena associated with intense heat. Cause and effect are demonstrably linked; the intense heat directly impacts the atomic and molecular structure of the material, leading to changes in its properties. This relationship is evident across various disciplines, from metallurgy to astrophysics.
The importance of understanding material effects under "deep hot" conditions is profound. For instance, in metallurgy, high temperatures during smelting and forging alter the crystalline structure of metals, impacting their strength, ductility, and resistance to corrosion. In high-temperature applications like jet engines and nuclear reactors, materials must withstand extreme thermal stresses. The ability to predict and control these material effects is crucial for designing components capable of withstanding such environments. Similar considerations apply to the study of stellar interiors, where intense heat fundamentally alters the composition and behavior of matter. In essence, understanding how materials react to extreme heat is a prerequisite for safe and efficient design in various fields. Real-world examples range from the heat treatment of steel for improved mechanical properties to the development of advanced alloys for aerospace applications, to the design of thermal protection systems for spacecraft re-entry.
In conclusion, "material effects" are intrinsically tied to "deep hot." Intense heat induces significant changes in the structure and properties of materials, fundamentally altering their behavior. Accurate predictions and controlled responses are vital for a range of applications, including industrial processes, engineering design, and scientific research. Challenges remain in predicting and managing these effects under extreme conditions, especially with novel materials. However, continuous research advances the understanding of material behavior under "deep hot" conditions, which, in turn, facilitates the development of more durable, efficient, and safe technologies.
6. Safety Protocols
Safety protocols are paramount when dealing with "deep hot" environments. The inherent risks associated with extreme temperatures necessitate comprehensive measures to prevent accidents, mitigate hazards, and ensure the well-being of personnel and the surrounding environment. These protocols encompass a spectrum of considerations, from material selection to emergency procedures, ensuring responsible and controlled operations.
- Material Selection and Design
Appropriate material selection is fundamental. Substances resistant to high temperatures, capable of withstanding thermal stresses, and possessing high melting points are crucial for constructing equipment and structures in "deep hot" environments. In metallurgy, selecting alloys with specific heat tolerances is essential. Failure to account for material limitations under these conditions can lead to catastrophic component failures and significant risks. Appropriate design principles, such as reinforcement, heat dissipation, and structural integrity, further safeguard against thermal damage.
- Monitoring and Control Systems
Continuous monitoring of temperature, pressure, and other relevant parameters is indispensable for maintaining control and safety. Sophisticated sensors and data acquisition systems are employed to detect potential deviations from prescribed limits. Automated shut-down mechanisms triggered by exceeding safety thresholds prevent escalation of hazardous conditions, like overheating or runaway reactions. These systems ensure real-time awareness of critical values and the implementation of protective measures.
- Emergency Preparedness and Response Protocols
Well-defined emergency procedures are essential in anticipating and responding to potential incidents. Clear evacuation plans, safety guidelines, and emergency contact information reduce the impact of unforeseen situations. Rapid response teams trained in handling "deep hot" emergencies, including specialized equipment and techniques for containing accidents, are essential components of these protocols. Regular drills and simulations further enhance the preparedness of personnel and expedite the response time in critical situations.
- Personnel Training and Certification
Thorough training programs for personnel operating in "deep hot" environments are essential. Comprehensive safety protocols, emergency procedures, and hazard identification are paramount for effective implementation. Certified personnel, possessing specialized knowledge and skills, are better equipped to handle potential hazards. Regular refresher courses and ongoing training programs are critical to ensure ongoing competence and vigilance in potentially perilous situations.
In summary, effective safety protocols are integral to managing the risks inherent in "deep hot" environments. Careful selection of materials, sophisticated monitoring systems, well-defined emergency procedures, and personnel training are key components. These measures are fundamental to ensuring the safety and security of personnel, equipment, and the surrounding environment, thereby mitigating potential consequences and enabling the safe and controlled operation in extreme temperature conditions.
7. Scientific Investigation
Scientific investigation plays a critical role in understanding "deep hot" phenomena. The exploration of extreme temperatures demands meticulous study, pushing the boundaries of current knowledge and fostering advancements in various scientific fields. Investigative methodologies and empirical data are crucial for comprehending the complexities of materials under intense heat and for mitigating potential risks.
- Thermal Properties of Materials at Extreme Temperatures
Investigating how materials behave at high temperatures is essential. This involves determining thermal conductivity, heat capacity, and expansion coefficients. This data is vital for designing materials capable of withstanding extreme heat in industrial applications and for understanding geological processes like volcanic activity. Measurements of these properties are made through sophisticated experimental setups, involving high-temperature furnaces, advanced instrumentation, and rigorous analysis to ensure accuracy. Examples include studies of refractory materials used in aerospace applications and high-temperature superconductors, crucial for efficient energy transmission. Accurate data on material behavior is paramount for safety and optimization.
- Modeling and Simulation of Deep Hot Systems
Mathematical models and simulations aid in comprehending intricate systems involving intense heat. Numerical modeling allows researchers to predict the behavior of materials and processes under extreme temperatures when real-world experimentation is challenging or impractical. This includes simulations of nuclear fusion reactions and the interiors of stars, where conditions are too extreme for direct observation. Modeling provides insights into energy transfer, reaction kinetics, and material transformations, aiding in the optimization of industrial processes and the development of improved technologies. Verification and validation of these models are essential, often achieved through comparison with experimental data and independent analysis.
- High-Temperature Chemistry and Phase Transitions
Understanding how chemical reactions and phase transitions occur at elevated temperatures is vital. This investigation delves into the effects of intense heat on molecular structures and chemical bonding, revealing the mechanisms governing phase changes, decomposition, and synthesis under these conditions. This knowledge aids the development of new materials and processes in areas like catalysis and industrial chemistry. Examples include research on novel high-temperature catalysts or the behavior of specific materials in extreme environments. The results facilitate the optimization of processes and the understanding of underlying chemical mechanisms.
- Instrumentation and Measurement Techniques for Extreme Environments
Developing tools and methodologies to accurately measure and monitor conditions in high-temperature environments is critical. This includes the creation of specialized sensors, high-temperature furnaces, and innovative measurement techniques. Precise and reliable measurements under these conditions are vital for data collection, establishing correlation, and verifying models. Examples include the development of optical pyrometers for remote temperature sensing or advanced techniques for monitoring the properties of plasma in fusion reactors. This advancement enables more accurate observation, facilitating the understanding of "deep hot" phenomena.
In conclusion, scientific investigation is indispensable for addressing the challenges associated with "deep hot" phenomena. From characterizing material properties to developing modeling tools, advancing measurement capabilities, and uncovering chemical processes, these efforts contribute to a deeper understanding of the universe. This rigorous approach supports advancements in industrial technologies, environmental protection, and the broader scientific community's comprehension of extreme environments. The continued pursuit of knowledge in this area is paramount for future technological advancements.
Frequently Asked Questions
This section addresses common inquiries regarding "deep hot," focusing on scientific, industrial, and practical aspects. Clear and concise answers are provided to enhance understanding.
Question 1: What constitutes a "deep hot" environment?
A "deep hot" environment is characterized by exceptionally high temperatures, often exceeding the melting points of common materials. These temperatures are present in various contexts, from the Earth's interior, to industrial processes, and even stellar interiors. The defining characteristic is the pronounced intensity of heat, impacting the behavior of matter and driving various phenomena.
Question 2: What are some practical applications of understanding "deep hot" processes?
Understanding "deep hot" processes is crucial for numerous applications, including metallurgy (e.g., refining and forging), energy production (e.g., nuclear reactors and geothermal power), and material science (e.g., developing heat-resistant alloys). This knowledge enables the design of safer and more efficient technologies, leading to advancements in various sectors.
Question 3: What are the safety concerns associated with working with "deep hot" environments?
Safety protocols are paramount in "deep hot" environments. Extreme temperatures pose significant risks to personnel and equipment. Inadequate safeguards can lead to accidents, injuries, and environmental damage. Rigorous material selection, monitoring systems, and emergency procedures are essential for mitigating these hazards.
Question 4: How do geological processes relate to "deep hot" phenomena?
Geological processes, like volcanism and plate tectonics, are directly influenced by "deep hot" conditions within the Earth's interior. The intense heat drives magma formation, fuels volcanic eruptions, and contributes to the movement of tectonic plates. Understanding these relationships allows for better prediction and management of geological hazards.
Question 5: What role does scientific investigation play in understanding "deep hot"?
Scientific investigation is vital for understanding "deep hot." Empirical research, modeling, and simulation techniques are employed to study material behavior under extreme conditions. This research aids in developing new materials, optimizing processes, and enhancing safety protocols for a range of industrial and scientific applications. Experimental and theoretical studies are critical for gaining deeper insights into the complexities associated with intense heat.
In summary, "deep hot" encompasses a wide range of phenomena with significant implications across diverse fields. Understanding the characteristics, applications, safety concerns, and scientific investigation required to study these intense conditions is critical for progress in various areas.
This concludes the FAQ section. The next section will delve into specific examples of "deep hot" applications in industrial settings.
Conclusion
This exploration of "deep hot" environments has illuminated the multifaceted nature of intense heat. The investigation encompassed its fundamental role in geological processes, its critical application in industrial settings, and its profound impact on material properties. Key findings underscore the necessity for rigorous safety protocols, advanced material science, and comprehensive scientific investigation to effectively manage and leverage the power of extreme heat. The article highlighted the intricate relationship between intense heat, thermal properties, and the behavior of materials, emphasizing the need for meticulous analysis in all domains. The importance of controlling and understanding "deep hot" phenomena is evident across a broad range of applications, from forging metals to predicting volcanic eruptions, thus impacting industries and scientific understanding.
The complexities associated with "deep hot" environments remain significant. Ongoing research and development are crucial to advance our understanding of material behavior under extreme conditions. Future efforts should focus on developing innovative materials capable of withstanding intense heat, refining predictive models for complex systems, and implementing advanced safety protocols to mitigate risks associated with the application of "deep hot" processes. The pursuit of knowledge in this area holds potential for breakthrough advancements in various sectors, offering considerable benefits to society.
You Might Also Like
Coolest Yololary Spiderman Videos!Unwrap Deliciousness! Ramen Advent Calendar 2024
John Candy's Last Words: A Look Back At His Final Moments
Best MKV Cinemas: Movie Listings & Showtimes
Kent McCord: The Latest News & Insights
Article Recommendations
- Ed Hochuli The Legendary Nfl Referee And His Impact On Football
- Did Trump Get An Award From The Naacp Uncovering The Truth
- Exploring Wwwjerseyexpressnet The Lucy Collection

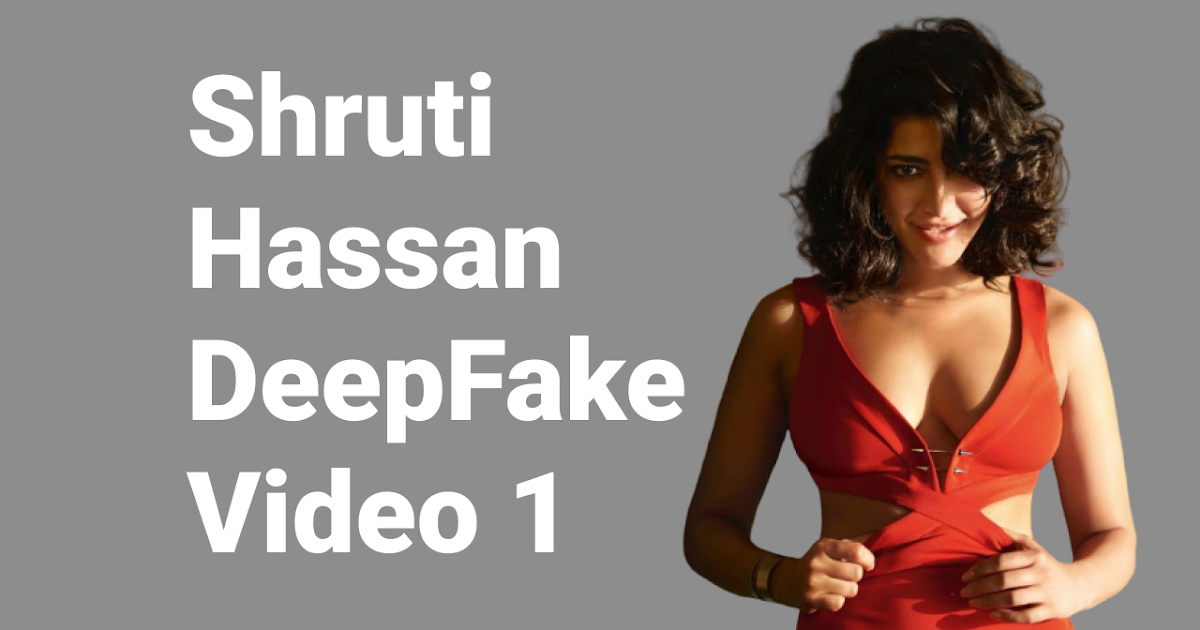
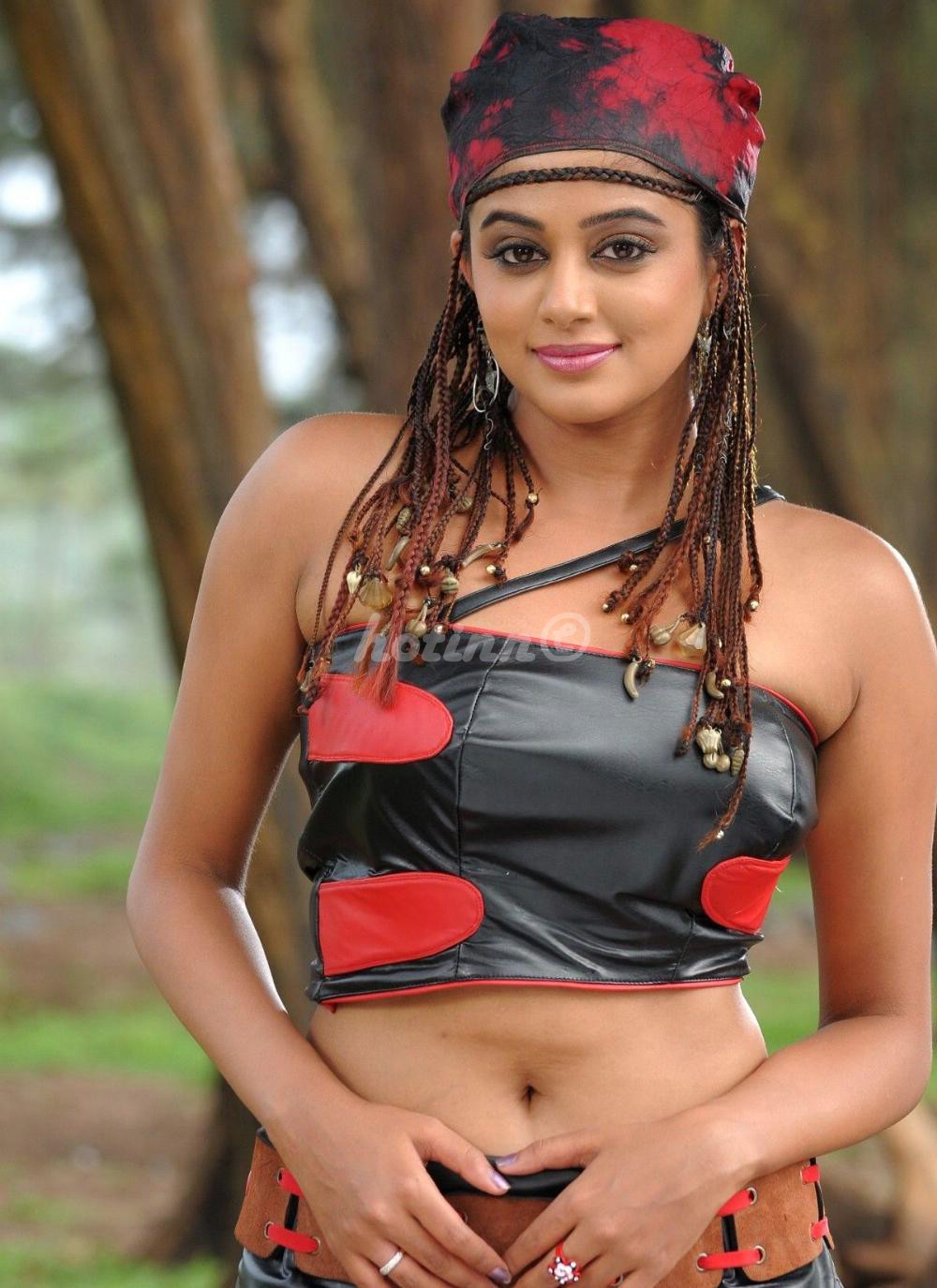